Mitocondrial pyruvate metabolism
Mitocondrial pyruvate metabolism(MPM) is a pseudo reaction that represents the total ATP production from one unit of pyruvate in the mitochondrian.
Contents
Chemical reaction

Rate equation
- Chemical reactions proceed to equilibrium within closed systems. For a simple reaction
it is defined as
where forward and reverse rates are equal.
- Equilibrium is not reached in open system due to influx and outflux. Mass action ratio[1]
for
reaction is defined as
where subscript ob represents observable at a given point.
- Deviation from equilibrium is measured with Disequilibrium constant
as
- Given the simple uni molecular reaction
the mass action equation can be modified as

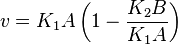
Considering and
we have,
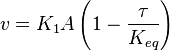
- The generalized arbitrary mass action ratio gives us
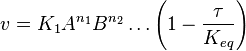

For eg. for the reaction , the rate law would be
- This equation demonstrates how a rate expression can be divided into parts that include both kinetics and thermodynamic properties [2].
- Given the net rate of reaction
, we have

In this model
- The rate law is defined as
- The overall standard free-energy change for Pyruvate metabolism is
[3][4]. Note The overall standard free energy is calculated by adding the standard free energy for all the reactions in TCA cycle.
- Calculating
value from these free energy gives
, Failed to parse (Cannot store math image on filesystem.): Keq = exp(-\frac{\Delta G'}{RT}) = exp(\frac{50300}{8.31*298.15}) \approx 654904512.15 . In order to ensure that the uncertainty does not affect the model equilibrium a small uncertainty of 5% can be considered for transporter. In our model we have applied this approach. So Failed to parse (Cannot store math image on filesystem.): K_{eq} = 654904512.15 \pm 32745226 .
- Calculating
- The Flux of pyruvate consumed by mitochondria measured for AS_30D is
[5].
- The steady state concentrations for substrates and products are
,
,
and
.
- The
value calculated from the above mentioned values are Failed to parse (Cannot store math image on filesystem.): 7.78E-015
- To calculate the uncertainty of
we first looked at the uncertainty on the substrate and product concentration. The maximum uncertainty reported for these values are
. In our model we considered this
uncertainty in its mean value giving value of
- Alternative The above mentioned formulate fails to reach steady state. So a constant flux mentioned in Hernandez et. al. can be used.
Parameter values
Parameter | Value | Organism | Remarks |
---|---|---|---|
![]() |
![]() |
||
![]() |
![]() |
Parameters with uncertainty
Parameter | Value | Organism | Remarks |
---|---|---|---|
![]() |
![]() |
||
![]() |
![]() |
References
- ↑ Hess B. and Brand K. (1965), Enzymes and metabolite profiles. In Control of energy metabolism. III. Ed. B. Chance, R. K. Estabrook and J. R. Williamson. New York: Academic Press
- ↑ Sauro H M, Enzyme Kinetics for Systems Biology, Second Edition, Ambrosius Publishing (2013), ISBN-10: 0-9824773-3-3
- ↑ Nelson D. and Cox M. (2008), Lehninger Principles of Biochemistry, Fight Edition, W.H. Freeman and Company, ISBN-10: 071677108X
- ↑ http://crystal.res.ku.edu/taksnotes/Biol_638/notes/chp_16.pdf
- ↑ Marín-Hernández A, Gallardo-Pérez JC, Rodríguez-Enríquez S et al (2011) Modeling cancer glycolysis. Biochim Biophys Acta 1807:755–767 (doi)